A history of the Solar system
purpose
This history differs from its contemporaries primarily in emphasis.
It emphasizes that it is possible to explain many properties of our present day Solar system by considering the mechanisms that were in play in the early Solar system. It is an accretion theory of the Solar system, and of the Earth-Moon system, and explains how the accretion theory accounts for the discrepancy in the chemical constitutions of the Earth and the Moon.
The early Solar system was a place very different from the one we know. Different forces were in effect. I hope to illustrate how several forces worked together to form the places we’re now familiar with. This picture is, I think, richer and more enlightening than collision theories that have recently become popular.
I think the calculation I do to suggest the density of the accretion disks is novel, albeit not very hard science.
formation of raw materials
The universe consists mostly of hydrogen and helium. However, the concentration of heavier elements in the present Solar system is greater than the average. Why might this be?
First, there was a big star. For a billion years, the process of nuclear fusion transformed its lighter elements into heavier elements. The energy released by this process provided heat that kept its mass suspended against gravitational collapse.
This process could not go on forever. Most heavier elements absorb more energy than they produce in the process of nuclear fusion. One day, there wasn’t enough of the lighter elements left to burn.
The core of the star underwent gravitational collapse. In a matter of seconds, gravity crushed it into very dense matter—a neutron star. This event released a tremendous amount of energy, which blew the remainder of the star out into space, enriched with its newly formed heavy elements.
There, the star’s gaseous remains cooled, and collided with other interstellar gas clouds, and went on to play other roles.
gravitational collapse
The gas clouds of our galaxy comprise a much larger part of the galaxy’s mass than do its stars and planets.
All matter causes gravitation, and gravity affects all material bodies in space. Since the gas clouds have mass, they exert gravitational attraction on other bodies, and upon themselves.
If gravitation were the only mechanism present, a gas cloud would quickly collapse under its own weight. However, there are two other mechanisms that act against such a collapse: bulk velocity, and pressure due to temperature.
If the various parts of a cloud are moving away from one another very rapidly, as would be the case after the explosion of a star, they may escape the overall gravitation of the gas.
The pressure of a gas can resist the force of gravity. If a gas cloud is too hot, it will tend to expand rather than contract under its own gravitation.
However, collision with another gas cloud, can reduce theier bulk velocity, and radiative cooling gradually reduces the temperature of a gas cloud.
Often, a galactic gas cloud will slow and cool so that it does collapse under its own weight.
formation of a disk
The orbits of the planets of our Solar system all lie within a few degrees of a perfect plane. Excluding Pluto, they are within 7 degrees of a plane. Excluding Mercury, they’re all within 3.5 degrees.
Furthermore, the planets of our Solar system all orbit the Sun in very nearly circular orbits. Once again, excluding Pluto and Mercury are all within 10% of perfect circles. Excluding Mars, they are within 6% of perfect circles. Earth’s orbit deviates from a perfect circle by only 1.6%—if you draw it on a page with a pencil, you can’t distinguish it from a circle.
On the other hand, once a planet is in an elliptical orbit, it tends to stay there. This is because the orbital motions of planets conserve energy. We have to conclude that the planets were born in nearly circular orbits about the Sun.
How did this familiar, planar, circular motion come to be?
The motion of a gas cloud under gravity is affected by mechanisms that are insignificant in systems of solid gravitating bodies. Primarily, these forces are due to gas pressure and friction. Moreover, electrostatic and magnetic forces come into play.
A gas cloud loses energy — it radiates heat due to friction. Angular momentum, however, must be conserved.
Gas moving in random directions within a collapsing cloud will tend to lose energy, and to fall into a configuration that has the least friction while maintaining angular momentum: a rotating disk.
Any portion of gas orbiting the center of gravity of the cloud, but not in the common disk, will collide with gas in the disk, and lose energy in that collision. The energy will be radiated as heat into space, and the average motion will become more planar and circular.
Out of the gas cloud, an accretion disk forms, a proto-planetary system. The form of the disk is familiar: like a spiral galaxy, it is round and flat with a central bulge.
This is why the planets of the Solar system almost all lie within a few degrees of a perfect plane, and within a few percent of circular orbits about the Sun. They inherited their co-planar, circular orbits from the circular motion of the gas in the accretion disk that was the proto Solar system.
Furthermore, the fact that the planetary orbits are still very circular suggests that no star-sized object has come near our Solar system since it first formed.
angular momentum
Angular momentum is the measure of the tendency of a rotating body to remain rotating. Angular momentum is always conserved.
For more information on angular momentum, see Angular Momentum in the Solar System.
Where is most of the angular momentum in our Solar system?
More than 96% of it is in the orbit of the planets about the Sun; only a few percent is in the rotation of the Sun itself. In fact, more than 60% of the angular momentum of the Solar system is in the orbit of Jupiter alone!
If the Sun were made to spin fast enough to have this angular momentum, it would be torn apart by centrifugal forces.
This is why almost all stars must have companions, either planets or other stars. The angular momentum of an immense gas cloud cannot be concentrated into the relatively tiny radius of a star.
Likewise, most planets will have companions.
proto-bodies
An accretion disk, as we’ve described it, isn’t stable. Even if there were a perfectly smooth, spinning, disk-shaped object, it couldn’t stay that way for long. The inner areas orbit the center faster than the outer areas. This causes vortexes in the disk, which tend to divide the disk into rings.
A vortex can become a proto-planet (or proto-moon), with its own accretion disk forming from the material in the neighboring bands.
Here’s a nice computer-generated picture of a proto-planet feeding off such bands
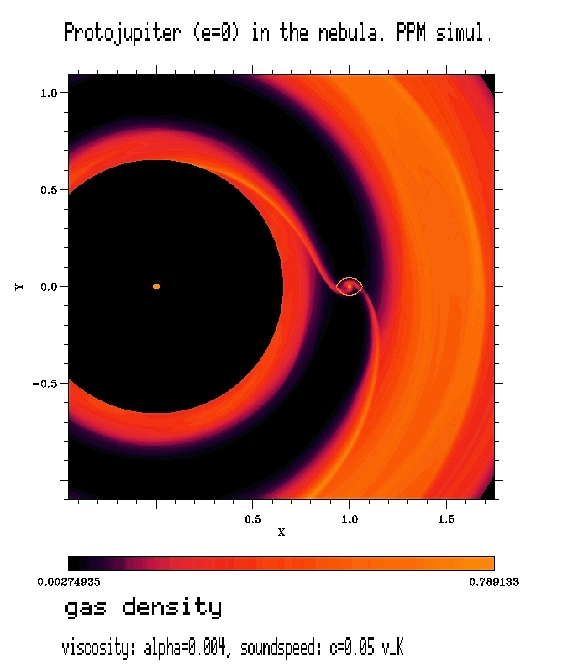
From Mass Flow and Accretion through Gaps in Accretion Discs by W. Kley.
accretion
Where the temperature is low enough, chemical molecules form when atoms in the cloud collide and adhere to one another. These molecules can then collide to form crystals (a “dust”). The crystals then collide to form aggregates of crystals, and finally larger bodies, proto-planets, a proto-Sun, a proto-Earth and a proto-Moon, all floating in a gas.
In just the right conditions of pressure and temperature, there can even be liquid water, and other liquids.
It’s a mélange.
As gravity compresses the gas, these chance collisions become more likely, and the process accelerates.
As accretion progresses, mass becomes more concentrated. However, the cloud can’t collapse immediately, because the compression would generate too much heat, which would cause the gas to expand. It has to gradually radiate heat in order to collapse.
Nearby areas in the cloud usually consist of similar elements. The degree of similarity depends on how homogeneous the initial gas cloud was.
In the presence of an accelerating fluid, materials can easily become separated by weight. The swirling gas clouds about a proto-star or proto-planet do not rotate as a solid disk: the inner material orbits faster than the outer material, This produces friction, and pressure waves that move through the gas.
While the gas and dust is suspended in this state, partly by gas pressure, gravity tends to sort the elements by weight. Thus, in particular, the Earth eventually acquires more iron and other heavy elements than does the Moon.
In this period, the nuclear fires of the Sun have not started to burn. The temperature in the cloud is due mostly to the compression of the gas, and friction as it rubs against itself.
the density of accretion disks
Let’s do a rough calculation to get a hold of how dense the Earth-Moon accretion disk might have been.
Air is a gas that we’re all familiar with. How big of a disk made of air weighs the same as the Earth? Let’s say the thickness of the disk is the same as the diameter of the Earth.
Consider these facts:- The density of air (at “Standard Temperature and Pressure”) is 1.2250 kg/m3.
- The average density of the Earth is 5515 kg/m3, about 4502 times denser than air.
- The square root of 4502 is about 67.
Therefore, a flat disk of air with the thickness equal to the diameter of the Earth and mass equal to that of Earth has a radius about 67 Earth radii.
Compare this to the present distance of the Earth to the Moon: about 31 radii.
We think of an accretion disk as being thin in some parts, bulgy in others, not of uniform thickness—rather like the spiral galaxies we see in the sky. Much of its material was likely in the form of solid (or liquid!) chunks. That would tend to reduce the average gas pressure. On the other hand, the bulk of the lighter gasses in the Earth-Moon system were probably blown away by the Sun’s initial winds (next section). This additional material would have tended to increase the average gas pressure.
At some point, the typical density of a disk smaller than 67 radii might have been much greater than that of air.
In the present-day solar system, the density of the gas in interplanetary space is very low. It also consists primarily of the multi-million-degree solar wind, streaming out from the Sun. Our nearby space is in effect inside the hot, sparse bubble of the Sun’s extended atmosphere. Before the Sun’s nuclear fires ignited, however, the environment was very different.
Could you have strapped on a pair of wings and flown to the Moon?
Material literally rains down out of the cloud onto the proto-planets, falling through this substantial gas. Chunks, dust, crystals, and even droplets of water, condense and fall.
Sun density:
1441 kg/m3
Radius is 109 times that of Earth (about 436 thousand miles):
An air disk the thickness and mass of the Sun would be about 38 Sun radii.
Earth orbits at about 220 Sun radii.
The Sun fires up
Why is it that the outer planets, excluding Pluto, are huge “gas giants”, while the inner planets are all relatively small and rocky?
Very young stars are typically much hotter than older stars of similar size. In the first four million years or so of a star’s life, the heat from gas compression supplements heat from nuclear fusion. This is called the T Tauri period (after a star that is currently in such a state).
Some estimate that, when it was going through its T Tauri period, the Sun was four times as bright as it is currently. See this article “New model shows sun was a hot young star” on Günther Wuchterl’s research.
The heat of the present-day Sun would have been sufficient to blow away what remained of the accretion disk of the proto Solar system. The T Tauri heat would have blown away most (or all) of the atmospheres of the inner planets as well.
The inner planets were originally more like gas giants.
Before the Sun ignited, the inner proto-planets all had thick gaseous mantles. In the case of Earth, it would have been arbitrary to distinguish this mantle from its accretion disk, which, at some point, reached beyond the Moon.
By comparison, the present-day “gas giant” planets Uranus and Neptune, while 17 and 14 times the mass of Earth, presumably have rocky cores comparable in size to Earth.
It is possible that all the atmosphere of the Earth was blown away by the early Sun. The present day Earth’s atmosphere and oceans can be accounted for by out-gassings from the remaining rocky core (the mass of the Earth’s oceans is something less than 1/10000 the mass of the planet).
Much of the remainder of the Solar system’s accretion disk escaped the present system, blown off into interstellar space by the Sun. However, some still lurks on its fringes (a region named the Kuiper belt) in the form of very cold ice balls. These ice balls sometimes become comets. Nowadays, it is popular to consider the planet Pluto as a nearby Kuiper belt object rather than a distant planet.
Nowadays, the notion of accretion disks is much more than theory. There are photographs of nearby stars which seem to show very plainly disks of dust and gas:
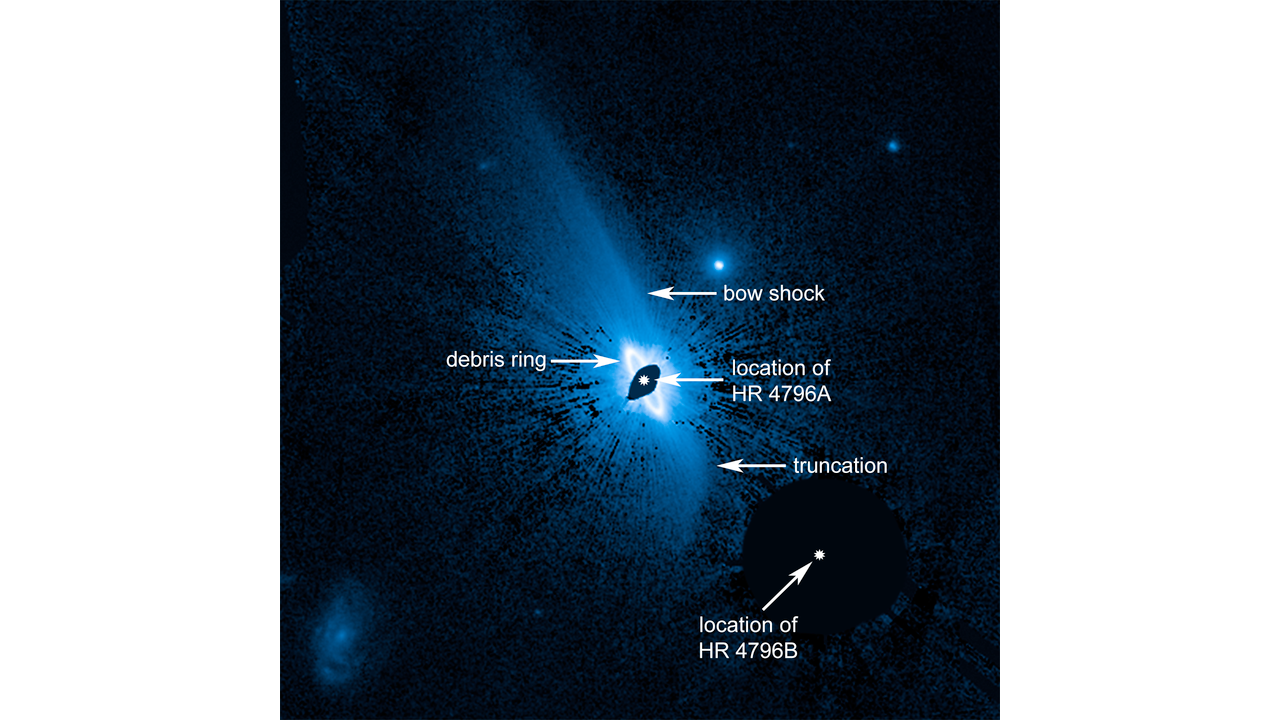
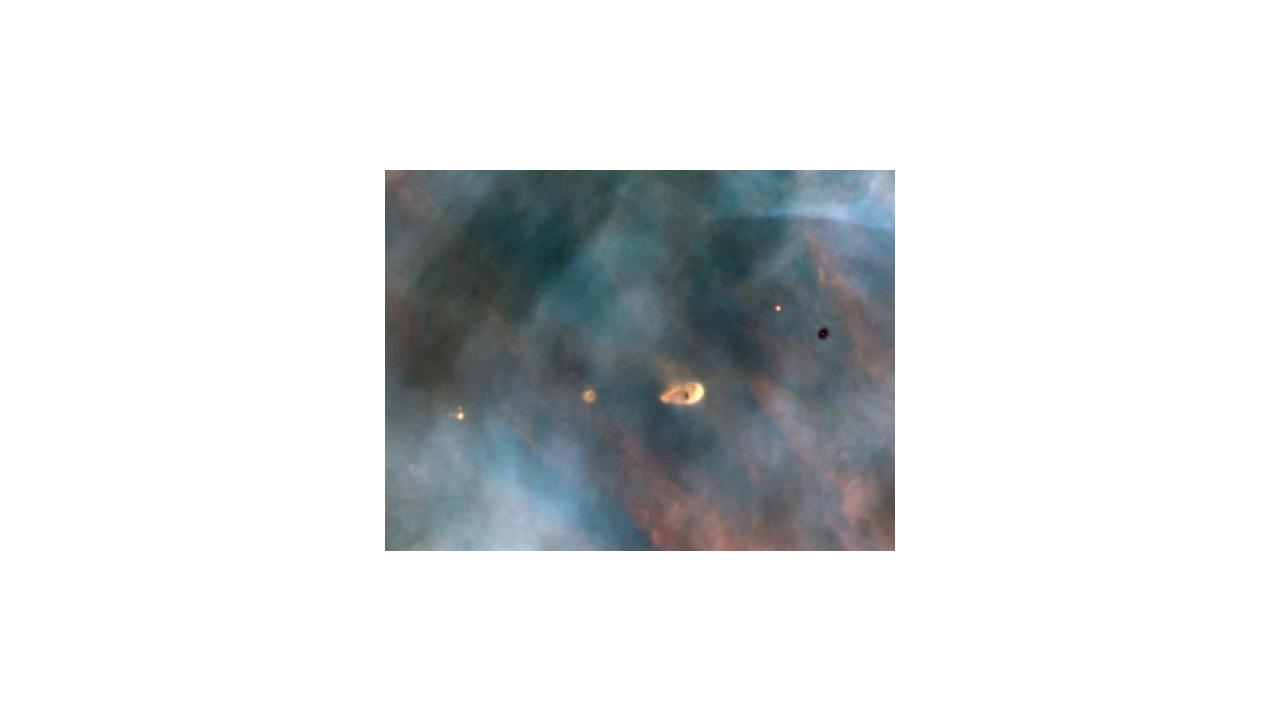
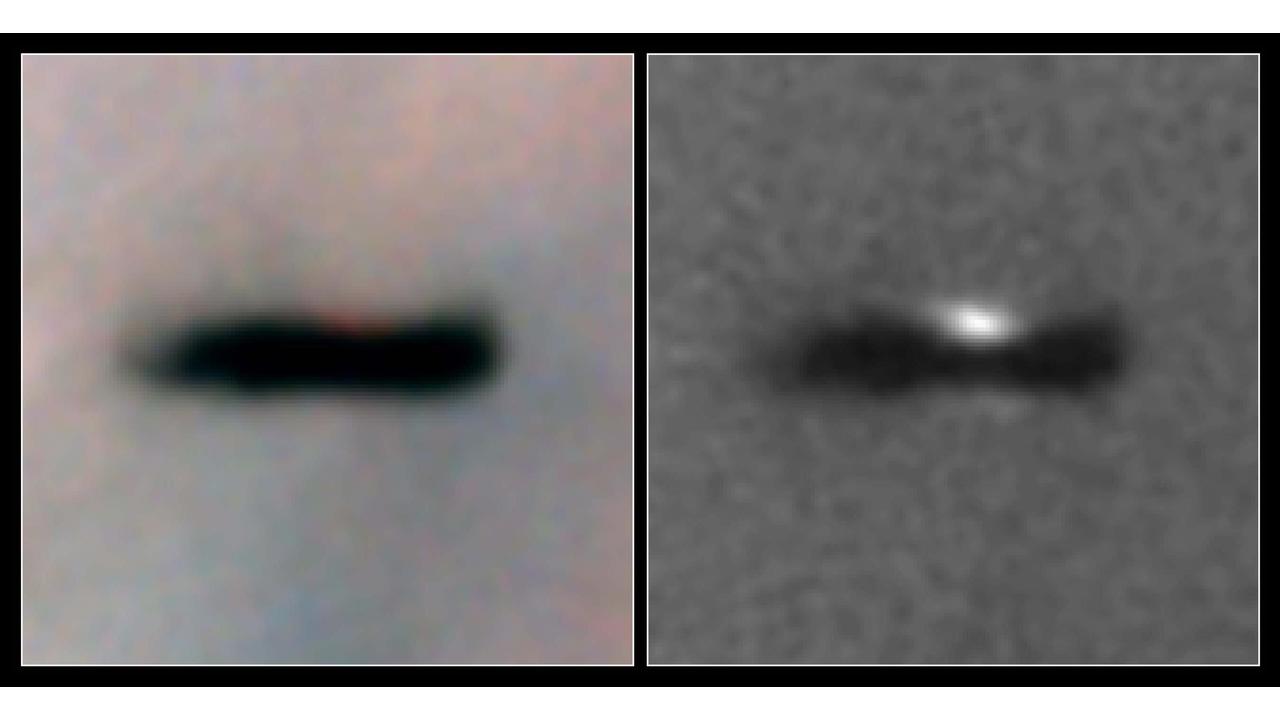
Some photos of proto-planetary disks by the Hubble Space Telescope. Stars with accretion disks. There are many more..
the early days
The planets cool and suffer a continued bombardment by meteors.
Jupiter remains very hot on its own; it cooks its inner satellites.
Mars has a substantial atmosphere!
Earth develops a thick atmosphere of carbon dioxide and water vapor. That will change soon, by processes that are not considered part of Astronomy. The Moon is initially half as far away from Earth than it is presently, and rotates much faster than it does now.
Today, the asteroid belt between Mars and Jupiter contains enough matter for a small planet (smaller than the Moon). It is an open question whether it is the remains of a destroyed planet, or that it is the remains of a planet that could never form because of the tugging effect of Jupiter.
My opinion on this was strongly influenced by pictures of the asteroid Eros (animation Feb 17, 2000) taken by the NEAR space probe. This object is concave. I cannot conceive of how such a thing could have been formed by gravitational means. To me, it looks like a shard of something that was once solid.
the present state
Our Moon presents only one of its hemispheres (its “near side”) to us. It rotates on its axis exactly once every time it revolves around the Earth.
This is not unusual in the Solar system. Most of the moons of the Solar system’s planets present only one hemisphere to their planets.
This comes about due to “tidal” forces.
Bombardment by asteroids much slower than it once was.
The Sun, now composed of about 3/4 hydrogen, 1/4 helium, has several billion years left.
The “heliopause” where the Solar wind ceases to blow.
Related issues: the orbital periods of the moons of Jupiter are integer multiples of one another, the apparent stability of the rings of Saturn and the asteroid belt, odd rotational periods of Mercury and Venus.
the future
Earth’s rotation slows, due to tidal forces of the Moon. Finally, it presents just one face to Moon, which is twice as far away as it is now. The dynamics were published by George Howard Darwin in 1879. See biography.
When the Sun has burned its hydrogen fuel, it will start burning helium and other elements, but will become a “red giant” star as part of the process. The inner planets will all be cooked. Then when it has burned all its helium, it will go explode as a “nova”. Finally, it will quiet down to a cinder, a “white dwarf” star.
Possibly some star comes too near the Sun, and all the Suns planets will be lost to cold interstellar space.
Earth and biology
The biology of the Earth touches this astronomical story in several ways.
First, the oldest rocks on Earth (and the Moon) are about 5.5 billion years old. This is taken to indicate when the planet first solidified. The oldest biological fossils to date, are about 4.5 billion years old.
Life is nothing new on planet Earth. It came into being shortly after the planet’s formation, not long (in geological time) after conditions became favorable for life to exist. (Stephen Hawking pointed this out in a talk I attended in Vancouver, B.C. in the mid ’90s.)
Next, the atmosphere of the Earth is one quarter molecular oxygen, whereas the atmosphere of no other planet in our Solar system contains free oxygen. Oxygen is very reactive, so this situation would be impossible without some chemical pump keeping the oxygen free. That chemical pump is the photosynthesis of plant life.
Does the existence of life imply the existence of free atmospheric oxygen? No, but such a chemical imbalance would be an important clue that life exists on a planet.
Finally, a well-regarded theory explaining the extinction of the dinosaurs, as well as several earlier extinctions, involves a collision of the Earth with relatively small asteroids. Life on Earth is normally placid only because most of those asteroids left over from the formation of the Solar system have been swept up by the Sun or planets or ejected from the Solar system.
other theories
The accretion theory is very old. It can be traced back as far as René Descartes in 1644, and has been propounded by such notables as Kant and Laplace.
An alternate group of theories involve things colliding with one another or spinning so fast they blow up. These collision theories have gone in and out of fashion over the years.
At some point, the dividing line between the two groups is a little hazy. Accretion-type theories involve material falling into bodies, and most collisionists don’t deny that some accretion occurred.
The French naturalist Gorges Buffon put forth a theory of Solar system formation in his 1749 work Histoire et théorie de la terre, whereby a comet was to have struck the Sun, producing a plume of material that coalesced into the planets.
In 1796, the German philosopher Immanuel Kant and the French mathematician Pierre-Simon Laplace both (and apparently, independently) published theories of Solar system formation, based on a “nebular hypothesis”, that the Solar system formed from a cloud of material. (Both Kant and Laplace correctly argued that some of the astronomical nebulae seen in telescopes were in fact composed of stars, were “island universes”. This insight was never accepted by the scientific community until the 1920s, when it was proved by Edwin Hubble.)
In his Exposition du système du monde (1796), Laplace argues that the friction in a gaseous system provide the only mechanical explanation for the fact that the planets and most of the planets’ satellites orbit in the same direction, nearly in a plane.
George Howard Darwin (son of Charles), in 1878, proposed that the early Earth spun so fast that the Moon shot out of it. It should be noted that his work on tidal forces was very respectable.
There are serious mathematical problems with this idea, but the overriding objection for me is: How did the Earth come to be spinning so fast in the first place?
A recent theory called Big impact was supposedly invented to explain the high angular momentum of the Earth-Moon system, and why the Moon is composed of lighter materials. It invokes a “rogue” planet crashing into an early, moonless Earth, knocking out enough material to create a dust cloud which then accretes(!) to form the Moon.
As to why the angular momentum is a problem, I have no idea how they could have made such a judgment—there are so many variables involved.
Of course, the accretion theory easily accommodates the sorting of elements by weight. In the above account, I have stressed that accretion disks are largely gaseous, and that their dynamics are significantly influenced by gas pressure. Those who oppose the accretion theory on the grounds of that it doesn’t explain the Earth-Moon chemical differentiation invariably describe accretion disks as being composed of dust, which by tacit assumption obeys only gravitational dynamics—not gas dynamics. Such a scenario would not have led to differentiation, but it could not have been the case in our Solar system.
The main reason that researchers have assumed that the Earth accreted from a dust cloud was that it is supposed that the inner Solar system was very hot at the time of the Earth’s accretion, that most of the lighter materials had already evaporated.
The Big Impact theory doesn’t explain why the Earth’s orbit is nearly circular, and why the moon’s orbit is so near the plane of the other bodies in the Solar system. It is unlikely that a rogue planet would strike the Earth in such a way as to not only knock out material to produce the Moon, but also to knock it into a circular orbit, in just such a plane. Moreover, it amounts to a logical regress: If it is impossible for two bodies of different constitution to accrete from the same gas cloud, then where did this third body come from? It couldn’t have come from the same gas cloud as our Solar system, or it would have to have the same constitution as the Earth!
And… where is the rogue planet now? And why the insistence that a single impact is to blame? Why not two? If two, why not millions? It seems to me that there must be some emotional appeal for the collision theory: maybe just the picture of big things going boom.
The big distinction between the collision theories and accretion is that collision theories propose something extraordinary to explain orbital situations, while the accretion theory proposes that the orbital situations we see in the Solar system are ordinary. The former is exciting, but isn’t very enlightening about typical solar system formation. Once we are able to resolve planets and their moons orbiting other stars, this issue will be resolved.
A psychiatrist named Velikovsky published a book called “Worlds in Collision”, in which he interprets various ancient myths as proof that Venus popped out of Jupiter, had various affairs with other planets, had a brief fling as a comet, then matured and settled down in a nice circular orbit.
Maybe she’s pinin’ away for the rogue planet.
Stuff found on Web:
Heeke’s “Shaker Effect”
NASA History site has Evolution of the Solar System